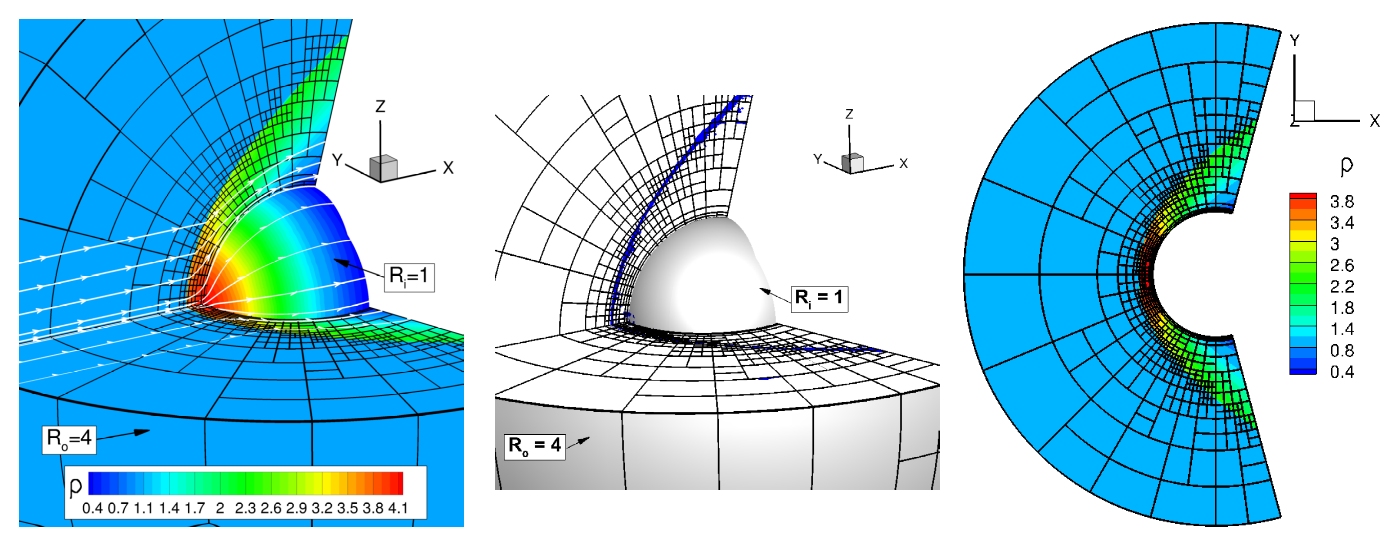
Accurate, Robust, and Scalable Computational Methods for Large-Scale Simulations of Multi-Scale Physically-Complex Flows
The routine solution of multi-scale, physically-complex flows for practical applications in a predictive manner necessitates significant future advances in numerical methods and CFD algorithm design. Toward this end, research is continuing in the CFD and Propulsion Group on the development and application of a range of novel, accurate, efficient, and robust adaptive solution methods for multi-scale physically-complex flows using new and emerging high-performance computing (HPC) architectures. This research includes the development of: (i) high-order spatial (both finite-volume and novel flux reconstruction approaches) and temporal discreatization schemes; (ii) anisotropic body-fitted and hybrid (the latter involves a combination of multi-block body-fitted and more general unstructured grids) adaptive mesh refinement (AMR) meshing strategies with local solution-dependent refinement following from output-based error estimates; and (iii) efficient and highly-scalable parallel algorithm design for effective use of future heterogeneous HPC multi-core systems with floating-point accelerators.
Funding/Partners: Natural Science and Engineering Research Council (NSERC), Digital Research Alliance of Canada